Yellow fever stops at the Miami airport.
Initially, new infectious diseases could spread only as fast and far as people could walk. Then as fast and far as horses could gallop and ships could sail. With the advent of truly global travel, the last five centuries have seen more new diseases than ever before become potential pandemics. The current reach, volume and speed of travel are unprecedented, so that human mobility has increased in high-income countries by over 1000-fold since 1800. Aviation, in particular, has expanded rapidly as the world economy has grown, though worries about its potential for spreading disease began with the advent of commercial aviation. [1]
Podcasts are great. But in the world of science podcasts, many are simply boring because there is only one person talking, or one person interviewing another person. Unless it’s slickly produced and edited (the Nature or Science Times podcasts), I’ll quickly lose interest.
It’s better when the podcast is three or four people who know each other, having a conversation. This is is the format of the TWi[X] series, particularly the flagship This Week in Virology. TWiV co-host Dickson Despommier, though not a virologist, contributes a big-picture point of view when the show leaves its territory of basic lab science and moves into epidemiology and patterns of disease outbreaks. Here’s his lecture on how West Nile arrived in North America and spread from state to state, energized by a very hot summer at the Bronx Zoo.
More than once I recall Dr. Despommier pointing out that though it’s all well and good to model how a epidemic might spread by looking at the day-to-day movements of people and mosquitoes, the most powerful and mobile disease vector is… the airplane.
That fact has become ever more clear with the SARS and West Nile outbreaks, as we used genetic analysis to track them across the continents in real time. But you might be surprised at how long ago people recognized the airplane as a challenge to disease control.
* * *
80 years ago, yellow fever was the archetypal mosquito-transmitted disease. Particularly as the disease was endemic in some parts of the world (e.g. South America), but not in others (e.g. North America), despite both places being home to the vector, Aedes genus mosquitoes. A 1934 article [2] coauthored by Henry Hanson, veteran of battles against yellow fever on three continents, points out that dengue fever (also transmitted by Aedes) had reappeared in Florida after an eleven-year absence, and yellow fever could do the same at any time.
Hanson and coauthor T. H. D. Griffitts go on to chide the cities of Florida and Georgia for their complacency in providing countless unsupervised water vessels in which the mosquitoes can reproduce.
Practically every urban community in the South has its array of artificial containers, from flower vases to catch basins, cuspidors and discarded automobile tires, producing Aedes aegypti. For example, the city of Tampa in eight weeks reported finding 1,091,823 containers (potential mosquito “hatcheries”). Of these, larvae were found in 20,864, or approximately 2 per cent. This was an unusually dry season (average rainfall of 1 1/2 inches a month for this period). It is interesting to note that in Miami for a like period only 56,598 potential breeding containers were reported, with 38,401, or 68 per cent, of the total actually breeding.
So I made an error above. The vessels aren’t quite “countless”. There are 1,091,823 of them in Tampa, give or take a dozen.
Though concerned that yellow fever could reach North America (again), Hanson and Griffitts are more concerned about India. The disease is only known in jungle areas of Brazil, Colombia and Bolivia (they claim); by comparison, Africa is its ancestral “home” and India, fairly nearby by plane, is virgin territory for yellow fever epidemics.
Today there is a feeling of concern … that Old World territory, where the vectors abound and where yellow fever has never before stalked, may experience widespread and devastating epidemics. One infective mosquito traveling in an airplane from the home of yellow fever (Africa) to India could be the spark to start the conflagration.
Thus there was considerable surveillance for mosquitoes and infected passengers at airports worldwide.
The earliest article I can find specifically discussing airplanes as disease vectors is from 1930, the first [3] of two identically titled 1930s editorials in the American Journal of Public Health. I don’t know if the international rules discussed here were enacted, but they show awareness that quarantine and disinsectization measures developed for ocean travel need to be multiplied and intensified to cope with passenger aircraft. Click for a bigger view.
* * *
For the purpose of determining whether or not mosquitoes are carried in airplanes, and, if so, to what extent, the distance of such transportation, the species of mosquitoes, and the type of planes on which they are carried, the United States Public Health Service began, on July 23, 1931, the inspection of all airplanes from tropical ports arriving at the airports of the Pan American Airways System at Miami. [4]
An anecdote from less than a year later illustrates how the search for mosquitoes had become a normal part of a plane’s arrival in the U.S. from South America.
The story of “the first international aerial hitchhiker” is as follows.
Paul Kaiser, 25, tried to immigrate from Czechoslovakia to America via a circuitous route. First, he got to the German port of Bremen, from which he sailed to Colón, Panama on an ocean liner. Sneaking into the nearby Canal Zone airport, he climbed into the baggage compartment of a “big 22-passenger Commodore plane”. The plane first landed in Barranquilla, Colombia, where he was not detected. It then landed in Kingston, Jamaica, where he was not detected. Finally, after Kaiser had spent 2 days without food, he landed in Miami. However, in Miami “a very thorough inspection is given every plane for mosquitoes, for there is always danger of the deadly yellow fever mosquito surviving the short trip and landing in the United States, a most undesirable immigrant”. Check out the April 1933 issue of Flying magazine for more details.
A few months earlier, T.H.D. Griffitts (coauthor of the aforementioned Henry Hanson article) performed the first experiments on mosquito transport by aeroplane. These were published in late 1931, in an enjoyably conversational article in Public Health Reports [4].
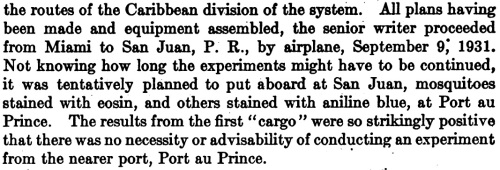
Dr. Griffitts, you don’t really have to describe the experiments you WANTED to do but then decided were unnecessary.
T.H.D. Griffitts (and J.J. Griffitts — his son?) start out by describing all the mosquitoes observed on normal commercial flights between July and September of 1931. Most were Culex quinquefasciatus, but several other species were observed including Aedes aegypti. In fact, a later Griffitts paper [5] indicates that the historic first mosquito discovered on a Miami-bound plane was Aedes aegypti. (It’s somewhat confusing that the insect apparently arrived on a “ship from San Salvador, El Salvador”, but I think that at this time the word “ship” could be used for aircraft. And San Salvador is definitely not a port city.)
Griffitts also put mosquitoes on three planes departing from San Juan, Puerto Rico, and looked for them upon arrival in Miami. Today it takes 2.5 hours to fly from SJU to MIA, but in 1931 they stopped at three other airports along the way and the average travel time was over 10 hours. A total of 100 mosquitoes were labeled with eosin dye (to distinguish them from non-experimental and Miami-resident mosquitoes). 22 were observed upon arrival in Miami, after 1,250 miles of flying, opening of doors and hatches, loading and unloading of luggage, etc. It seems obvious that the insects can be imported… but these experiments prove it.
* * *
Eight years after 1930’s “The Airplane and Yellow Fever” editorial, the American Journal of Public Health published another one [5], describing the policies for infectious mosquito control in Khartoum, Miami, and “the French Colonies and Mandates”.
As the 1930s progressed and India remained devoid of yellow fever, public health doctors kept amplifying their alarms about how devastating such an epidemic would be. From the 1938 editorial:
75 years after that was published, yellow fever still hasn’t swept through India.
In 1938, the number of people who’d been vaccinated against yellow fever was less than a hundred thousand, mostly in Brazil. It had taken a long time to make a vaccine strain of YFV that was weak enough that it could be given as a live vaccine, but the 1938 trial (run by the Rockefeller Foundation) was successful, and in 1942 the number of people vaccinated was over 10 million. For his two decades of work on the vaccine, which along the way required multiple basic science breakthroughs for it to be manufactured in large quantities, Max Theiler of Rockefeller University received the 1951 Nobel Prize in Physiology or Medicine. Read more here [6] about Theiler’s story; read more here [7] about the many problems and hurdles that were overcome to end up with a safe vaccine. (I think the second one is open-access and the first one isn’t.)
Within a decade, though complications from the vaccine were common and the virus stayed endemic in the tropics, Theiler’s YFV vaccine had eliminated yellow fever as a source of large-scale epidemics.
* * *
Coda: As early as the airplane was recognized as a vector for disease… it was harnessed as a weapon against disease.
From a 1932 report in Science (8), Joseph Ginsburg of the New Jersey Agricultural Experiment Station explains how large regions of standing water that previously were inaccessible to mosquito control workers can now be reached by plane, so that the surface can be coated with larvicidal pyrethrum or oil.
* * *
1. Tatem AJ, Rogers DJ, Hay SI (2006). Global transport networks and infectious disease spread. Adv Parasitol 62:293-343.
2. Griffitts THD, Hanson H (1934). Significance of an epidemic of dengue. JAMA 107(14):1107-1110.
3. Editorial (1930): The airplane and yellow fever. Am J Public Health 20(11):1221-1222.
4. Griffitts THD, Griffitts JJ (1931). Mosquitoes transported by airplanes: Staining method used in determining their importation. Public Health Rep0rts 46(47):2775-2782.
5. Editorial (1938): The airplane and yellow fever. Am J Public Health 28(9):1116-1118.
6. Norrby E (2007). Yellow fever and Max Theiler: The only Nobel Prize for a virus vaccine. J Exp Med 204(12):2779-2784.
7. Frierson JG (2010). The yellow fever vaccine: A history. Yale J Biol Med 83(2): 77-85.
8. Ginsberg JM (1932). Airplane oiling to control mosquitoes. Science 75(1951):542.